The therapeutic potential of circular RNA in triple-negative breast cancer
Abstract
Triple-negative breast cancer (TNBC) is among the most aggressive subtypes of the disease that does not express estrogen receptor, progesterone receptor, and human epidermal growth factor receptor 2. Circular RNAs (circRNAs) are a type of non-coding RNA with a circular shape formed by non-standard splicing or reverse splicing. Numerous circRNAs exhibit abnormal expression in various malignancies, showing their critical role in the emergence and growth of tumors. Recent studies have shown evidence supporting the idea that certain circRNAs regulate the proliferation and metastasis of TNBC. In addition, circRNAs alter metabolism and the immune microenvironment to promote or inhibit the development of TNBC. Notably, circRNAs may affect the efficacy of clinical drug therapy, serve as therapeutic targets, and be used as molecular biomarkers in the future. Herein, we will first summarize the biogenesis and function of circRNAs. Then, we will explain current research on circRNAs related to TNBC and their potential to serve as therapeutic targets or biomarkers for future drug development, providing a new direction and idea for TNBC therapy.
Keywords
INTRODUCTION
The latest research report indicates that breast cancer is now the most prevalent form of cancer in the world, with the highest incidence among all major cancers[1]. According to its histological characteristics and expression of estrogen receptor (ER), progesterone receptor (PR) and human epidermal growth factor receptor 2 (HER2), breast cancer can be subdivided into luminal A, luminal B, HER2 overexpression, and triple-negative breast cancer (TNBC), respectively[2,3]. The incidence of TNBC ranges from 15% to 20%[4] and it is difficult to identify the potential therapeutic targets. Thus, it leads to an increased risk of recurrence and metastasis and the patients have poor prognosis and shorter overall survival[5]. Investigation into the detailed molecular pathogenesis of TNBC and the development of potential therapeutic targets are important tasks in order to improve the prognosis more effectively and prolong the survival time of TNBC patients.
Circular RNAs (circRNAs) have recently gained significant attention as they are involved in various diseases, including human cancers[6] such as TNBC. Many studies suggested that circRNAs are strongly associated with the occurrence and development of tumors. They are non-coding RNAs that have been recently discovered and are formed by reverse splicing the precursor messenger RNAs (mRNAs). Compared with linear RNA, circRNAs do not have a 5 'end cap and a 3' end polyA tail which is more stable and not easily degraded by nuclease[7,8]. Its main functions include microRNA (miRNA) sponging[9], RNA binding protein (RBP) binding[10], and serving as a translation template[11]. Studies have indicated that circRNAs have the capability to regulate downstream genes and contribute to tumor proliferation, invasion, and metastasis. Therefore, identifying the specific role that circRNAs play in tumor development and occurrence is of great importance.
The first discovery of circRNA in eukaryotic cells was made through electron microscopy in 1979[12]. However, due to the scientific limitations at that time, circRNA was considered as a product of abnormal splicing events[13]. With the advancement of next-generation sequencing technology and bioinformatic analysis tools, a large number of circRNAs have been identified in eukaryotic genome and transcriptome[14]. This identification implies that circRNAs are not accidental by-products, but normal components of the body that are widely expressed in eukaryotes, including humans[15]. Therefore, scientists began to conduct detailed research on various functions served by circRNAs. Recently, an increasing number of circRNAs have been reported to be differentially expressed in breast cancer. These aberrantly expressed circRNAs mediate a range of tumorigenic processes, including cell proliferation, metastasis, apoptosis, and cell metabolism[16]. Hence, we summarized current information on abnormal expression of specific circRNAs that are implicated in TNBC development, followed by current clinical applications and potential therapeutic utility of circRNAs. It is clear that circRNA holds promise as a future therapeutic target[17].
FORMATION AND TYPES OF CircRNAs
The mechanism for circRNA formation is still not clear. It is generally accepted that they are derived from precursor mRNAs (pre-mRNAs), which are closed-loop molecules formed by back splicing[8]. Depending on their composition and synthetic mechanism, circRNAs can be categorized into three main groups: EciRNA, EIciRNA, and ciRNA[18]. First, EciRNAs are derived entirely from the exons of parental genes. In the process of forming EciRNA, a splice donor downstream of the 5' splice site is attached to a splice acceptor upstream of the 3' splice site, which is called back-splicing[15]. This molecular event produces a circular format of RNA with a 3'-5' phosphodiester bond at the back-splicing junction (BSJ) site. EciRNA is the most common type of circRNA and is mainly located in the cytoplasm[19]. Depending on the distribution of specific organelles, these circRNAs in the cytoplasm perform different functions. Second, EIciRNAs are circRNAs resulting from the retention of introns located in the 5' donor and the 3' acceptor on the
Figure 1. Biosynthetic mechanisms for circRNAs. (A) Lariat-driven circularization: lariat causes exons or introns that were originally far apart to come closer; (B) Intron pairing-driven circularization: complementary sequences in introns promote circRNA circularization by base pairing to bring the 3' splice acceptor site and the 5' splice donor site closer together in spatial conformation; (C) RBP-dependent circularization: RBPs bind to intronic sequences or specific motifs within flanking introns near the splice site and indirectly bridge the distance between upstream and downstream exons. RBP: RNA binding protein.
The vast majority of circRNAs have corresponding linear parental genes in organisms, which makes it difficult to be distinguished easily. Currently, the main approach is first to enrich circRNAs based on their unique stable structure by digesting total RNA with RNase R, and then to identify specific circRNAs through the detection of specific junction sequences by RNA high-throughput sequencing[29]. Although these methods can identify many BSJ sequences, their detection sensitivity is low once the BSJ sequence is very short. In recent years, some emerging long-read sequencing technologies have gradually developed, such as PacBio and Oxford Nanopore, which can better distinguish circRNA from its corresponding linear transcripts[30]. Furthermore, Chiang et al. have recently developed a FL-circAS nanopore long-read sequencing technology, which can decipher circRNAs from the aspects of their expression, formation, and function. This technology will promote the further development of circRNA research[31].
The FUNCTION OF CIRCULAR RNA IN TNBC
Sponging miRNA
In accordance with the base complementary pairing principle, miRNA inhibits or facilitates mRNA translation by binding to non-translational regions in target genes[32]. RNA that can competitively bind to common miRNAs to inhibit their activity on target genes is known as competitive endogenous RNA (ceRNA)[33]. Research has revealed that circRNAs carry a large amount of miRNA-responsive sequences, which can serve as effective ceRNAs. They bind to miRNA to adsorb it and effectively prevent its binding to the target genes, thereby providing regulatory effects on target genes[34].
Research on circRNAs in TNBC primarily concentrated on understanding the function of miRNA sponging. For example, CiRS-7 may be the most distinctive circRNA, containing over 70 conserved binding sites of miR-7, and has a stable expression in many tumors including TNBC[35]. Recent research shows that CiRS-7 also has miR-1299 binding sites besides miR-7. Experiments identified that downregulation of
Summary of miRNAs currently bind to circRNAs in TNBC
CircRNAs | Expression in TNBC | MiRNAs | Targets | Functions | Ref. |
CircCRIM1 | High | miR-503-5p | OGA/FBP1 | Increases glycosylation hydrolase OGA and decreases FBP1 | [37] |
CircEGFR | High | miR-1299 | EGFR | Regulates malignant progression of TNBC by controlling EGFR through miR-1299, leading to THP drug-resistant | [39] |
CircNCOR1 | Low | miR-638 | CDK2 | CircNCOR1 binds to hsa-miR-638 and targets CDK2 to regulate TNBC radio-sensitivity | [42] |
CircFGFR4 | High | miR-185-5p | CXCR4 | CircFGFR4 exerts an impact on immune evasion and resistance to PD-1 immunotherapy through miR-185-5p/CXCR4 axis in TNBC | [43] |
CircDUSP1 | Low | miR-761 | DACT2 | Reduces the suppression of DACT2 expression by miR-761 and thus increases the sensitivity of TNBC cells to paclitaxel | [40] |
Circ-TRIO | High | miR-432-5p | CCDC58 | Circ-TRIO may regulate CCDC58 expression by combining with miR-432-5p | [44] |
CircRAD54L2 | High | miR-888 | PDK1 | Regulates PDK1 expression by sponging the miR-888 family and PDK1 | [45] |
Circ0004676 | High | miR-377-3p | E2F6/PNO1 | Circ0004676 increases E2F6 and its target PNO1 expression by sponging miR-377-3p | [46] |
CircCSNK1G1 | High | miR-28-5p | LDHA | Circ-CSNK1G1 represses miR-28-5p and positively regulates LDHA expression | [47] |
CircUBR5 | High | miR-1179 | UBR5 | CircUBR5 upregulates UBR5 expression by suppressing miR-1179 | [48] |
CircDHDDS | High | miR-362-3p | DDX5 | CircDHDDS accelerates TNBC by upregulating DDX5 via miR-362-3p adaptation | [49] |
CircPRKCI | High | miR-545-3p | WBP2 and PI3K/AKT | CircPRKCI acts as miR-545-3p sponge to regulate WBP2 and AKT phosphorylation | [50] |
CircPTK2 | Low | miR-136 | NFBI and AKT/PI3K | CircPTK2 serves as miR-136 sponge and direct regulator of NFBI and AKT/PI3K pathways | [51] |
CircUBAP2 | High | miR-300 | ASF1B and PI3K/ AKT/mTOR | CircUBAP2 upregulates ASF1B by miR-300, which triggers (PAM) signaling to increase DDP resistance in TNBC. | [52] |
CircFAM64A | High | miR-149-5p | CDT1 | CircFAM64A sponges miR-149-5p to increase CDT1 expression and promote cellular processes in TNBC | [53] |
CircCD44 | High | miR-502-5p | KRAS and IGF2BP2/Myc | CircCD44 contributes to TNBC cell proliferation, migration, invasion, and tumorigenesis, in part through miR-502-5p sponge activity and interaction with IGF2BP2 | [54] |
Circ-PDCD11 | High | miR-432-5p | LDHA | By sponging up miR-432-5p, circPDCD11 enhances LDHA expression | [55] |
Circ-ERBB2 | High | miR-136-5p | PDK4 | As a result of miR-136-5p inhibition by circ-ERBB2, PDK4 expression is increased | [56] |
CircWHSC1 | High | miR-212-5p | AKT3 | CircWHSC1 promotes malignancy and glycolysis in TNBC cells by sequestering miR-212-5p. | [38] |
CircWAC | High | miR-142 | WWP1 and PI3K/AKT | CircWAC sponges miR-142 to elevate WWP1 expression and activate PI3K/AKT pathway | [57] |
CircNR3C2 | Low | miR-513a-3p | HRD1/ Vimentin | Overexpression of circNR3C2 significantly improves the tumor suppressive effects of HRD1 by sponging miR-513a-3p | [58] |
CircPSMA1 | High | miR-637 | Akt1/β-catenin (cyclin D1) | CircPSMA1 functions as a promoter of tumor growth through the circPSMA1/miR-637/Akt1-β-catenin (cyclin D1) regulatory pathway | [59] |
CircBACH2 | High | miR-186-5p /miR-548c-3p | CXCR4 | The expression of CXCR4 is increased, resulting from the sponging of miR-186-5p and miR-548c-3p by ircBACH2 | [60] |
Circ_102229 | High | miR-152-3p | PFTK1 | Hsa_circ_102229 could regulate the expression of PFTK1 by targeting miR-152-3p | [61] |
CircUBE2D2 | High | miR-512-3p | CDCA3 | CircUBE2D2 facilitates the advancement of TNBC and increases resistance to doxorubicin by functioning as a miR-512-3p sponge, which results in elevated CDCA3 expression | [41] |
Circ0000199 | High | miR-613 and miR-206 | PI3K/Akt/ mTOR | Hsa_circ_0000199 improves sensitivity to chemotherapy in TNBC by promoting the expression of miR-206/miR-613 and deactivating PI3K/Akt/mTOR signaling pathway | [62] |
CircIFI30 | High | miR-520b-3p | CD44 | CircIFI30 sponges miR-520b-3p, thus releasing the CD44 expression | [63] |
CircPGAP3 | High | miR-330-3p | Myc | Circ-PGAP3 promotes the development of TNBC cells by inhibiting miR-330-3p through its sponging function, leading to increased gene expression of the proto-oncogene Myc | [64] |
CircFBXW7 | Low | miR-197-3p | FBXW7 | FBXW7 gene sponges miR-197-3p and produces FBXW7-185aa protein to inhibit TNBC development by increasing FBXW7 expression | [11] |
CircPLK1 | High | miR-296-5p | PLK1 | CircPLK1 may be a target of miR-296-5p for the regulation of PLK1 expression | [65] |
CircKIF4A | High | miR-637 | STAT3 | CircKIF4A regulates STAT3 expression levels through miR-375 | [66] |
CircEPSTI1 | High | miR-4753 miR-6809 | BCL11A | CircEPSTI1 acts as a sponge for miR-4753 and miR-6809, which regulate the expression of BCL11A | [67] |
Binding to proteins
Results from a cross-linked immunoprecipitation experiment showed that circRNA interacts with various proteins[68]. The first study to demonstrate the function of protein binding was conducted on the splicing factor protein gene encoding muscle blindness (MBL) in Drosophila melanogaster, a homolog of human muscle protein 1. The gene produces circMBL in both Drosophila and humans, with the binding sites of MBL and MBNL1, respectively[69]. Similar to circMBL, other circRNAs in breast cancer also function by connecting with their target proteins. For example, circKIF4A stabilizes the mRNA expression of SDC1 by attaching to EIF4A, triggers the c-src/FAK signaling pathway, and leads to the advancement of disease in TNBC[70]. Mass spectrometry and RNA Binding Protein Immunoprecipitation experiments demonstrated that circSNX25 binds to COPB1(Coatomer Protein Complex, Subunit Beta 1) to promote the malignant progression of TNBC, bringing extremely poor prognosis[71]. The above two examples of circRNAs are intended to demonstrate their functions in binding to proteins. Additionally, circRNAs have the ability to facilitate mRNA translation. Circ_0076611 is a circRNA produced by MALAT1-dependent production that binds to many transcripts, including the mRNAs for MYC and VEGFA. It can affect the cell cycle and promote cell proliferation by facilitating the binding of MYC and VEGFA mRNAs to translation initiation factors and elevating their protein expression, leading to TNBC progression[72]. It also functions as a protein scaffold. For example, circEIF3H can directly combine with IGF2BP and HuR proteins to form the circEIF3H-IGF2BP2-HuR scaffolding complex, which in turn is inextricably linked to mRNA stability. In this case, circEIF3H promotes TNBC progression by stabilizing downstream HSPD1/RBM8A/G3BP1 mRNA expression in an indirect manner[73].
Encoding proteins
Recent research found that circRNA encodes proteins, which changes the traditional recognition that circRNA is non-coding RNA. Either the internal ribosome entry site (IRES)[74] or the m6 5' “untranslated region (UTR)” can be used for cell-independent translation of circRNA[75]. The protein encoded by circRNA can activate some downstream signal pathways to promote the occurrence of TNBC. CircFBXW7 can sponge miR-197-3p and encode a 185-aa protein, thus inhibiting malignant progression in TNBC[11]. CircCAPG produces a polypeptide known as CAPG-171aa. This polypeptide promotes cancer growth by inhibiting the binding between serine/threonine kinase 38 (STK38) and SMAD-specific E3 ubiquitin protein ligase 1 (SMURF1), which prevents MEKK2 from undergoing ubiquitination and proteasomal degradation[76]. Circ-EIF6 exerts pro-oncogenic effects on TNBC through its encoded peptide EIF6-224aa, which decreases the ubiquitinated degradation of the oncogene MYH9, thereby increasing its expression to activate the downstream Wnt/beta-catenin signaling pathway[77]. CircSEMA4B, which is significantly downregulated in TNBC tissues and cell lines, encodes a novel protein called SEMA4B. Both circSEMA4B and SEMA4B inhibit TNBC proliferation and migration in vitro and in vivo[78]. In addition, a study has shown that circHER2 is present in TNBC and encodes the novel protein HER2-103. This protein targets the HER2-targeting drug Pertuzumab, which has been commonly used in clinical treatment. It was suggested that some TNBC patients may benefit from Pertuzumab in the future[79]. However, so far, only a few circRNA has been found to encode proteins and the functional significance of most circRNA-derived peptides is still unknown.
THE ROLE OF CIRCULAR RNA IN TNBC PROGRESSION
The effect of circRNA in TNBC proliferation, metastasis and invasion
Circular RNAs have a crucial role in the malignant progression of TNBC, including cell proliferation, metastasis, and invasion. The common metastases of TNBC include brain, liver, lung, and bone metastasis, which ultimately leads to a high mortality rate due to the presence of lesion metastasis in different parts and degrees. Many circRNAs are highly expressed in TNBC, such as circRRM2[80], circ0000851[81], circEZH2[82], circ0042881[83], and circZFAND6[84] [Table 1]. A majority of them can enhance the miRNA sponge function, thus promoting the proliferation, migration, and invasion ability of TNBC, including circ0000851/
The role of circRNA in TNBC metabolism
Tumor experts consider metabolic reprogramming as one of the six fundamental hallmarks of tumor progression[87]. New research indicates that circRNA also plays a crucial function in tumor metabolism[88]. Currently, there is considerable research on the role of circRNA in TNBC metabolism, mainly focusing on glucose metabolism[89]. Circ-PDCD11 has been found to promote the progression of TNBC by enhancing aerobic glycolysis[55]. In addition, circ_0039960 is upregulated in TNBC cells, and knockdown of circ_0039960 significantly inhibits lactate production and glucose uptake, hinders the glycolytic process, and thus inhibits the progression of TNBC[90]. Many enzymes involved in glucose metabolism are regulated by circRNAs, such as hexokinase2 (HK2) and lactate dehydrogenase A (LDHA). Dou et al. reported that circ_0008039 was upregulated in TNBC tissue and cells, and knockdown of circ_0008039 inhibited TNBC cell proliferation, migration, invasion, and glycolysis. Western blotting demonstrated that knockdown of circ_0008039 can decrease the expression level of HK2, which was a key rate-limiting enzyme of glycolysis[91]. CircRNF20 also regulates glycolysis by affecting HK2[92]. Circ-CSNK1G1 regulates the glycolysis level of TNBC by acting on lactate dehydrogenase (LDH) through miR-28-5p[47]. Besides, circRNA derived from Myc promotes the progression of TNBC by reprogramming fatty acid metabolism. However, there is currently relatively little research on circRNA in lipid metabolism and others. A future advance is anticipated in our understanding of these topics.
The effect of circRNA in TNBC drug resistance
Currently, the primary method of treating TNBC in clinical settings involves chemotherapy, including doxorubicin, albumin paclitaxel, docetaxel, and platinum. Chemotherapy is the preferred option for TNBC and has benefited many TNBC patients. However, with the deepening of treatment, many patients have developed certain resistance to chemotherapy drugs, so their clinical use is still limited[93]. Chemo-resistant TNBC is a diverse and genetically unstable condition that presents a significant challenge to the application of personalized treatments[94]. Research has shown that circRNA plays an important role in drug resistance[95,96], such as circ_0000199. It plays a role in TNBC chemo-resistance through the AKT3/miRNA pathway. This work validates the circ_ 0000199 resistance to four chemotherapy drugs, including cisplatin, adriamycin, paclitaxel, and gemcitabine. The MiR-206/miR-613 inhibitor blocked the negative effects of
The effect of circRNA in TNBC immune microenvironment
Many circRNAs have been discovered in ovarian cancer[98], lung adenocarcinoma[99], colorectal cancer[100], esophageal cancer[101], pancreatic cancer[102], and oral squamous cell carcinoma[103] in which they regulate tumor immune microenvironment. CircRNAs can induce programmed cell death[104], and enhance PD-1/PD-L1 binding by increasing PD-1 expression, preventing T cell identification and triggering, thus causing immune escape of tumor cells. Moreover, circRNAs also regulate NK cells, macrophages, neutrophils, myeloid-derived inhibitory cells, and cancer-associated fibroblasts through complex pathways to affect tumor development. There are also some immune-related circRNAs in breast cancer. CircWWC3 induces repolarization of M1 macrophages to M2 by increasing the expression of IL-4 and PD-L1 that promotes the escape of immune cells from tumors and worsens the growth of TNBC. Breast cancer patients with high levels of circWWC3 or PD-L1 expression and high CD163-expressing macrophages are correlated with low overall survival (OS) and disease-free survival (DFS)[105]. Circ_0001142 was found to be highly expressed in BCs. It was packaged in exomes and released by endoplasmic reticulum stress which induces M1 macrophage repolarization and promotes TNBC progression through the PI3K/AKT pathway[106].
CircRNA and the clinical treatment of TNBC
Most tumor progression is due to a lack of early monitoring and examination of the body, and TNBC is no exception. Early detection and treatment can greatly improve the survival rate of TNBC patients. The structure of circRNA is stable and can be easily detected in blood, urine, saliva, and other biological tissues. Therefore, researchers are exploring the relationship between circRNA and tumor development and whether it can reflect the clinical prognosis of patients after treatment. Currently, many circRNAs have been found to be associated with the prediction, treatment, and prognosis of TNBC[107]. The relevant circRNAs identified in TNBC in recent years are summarized in Table 2. The vast majority of the circRNAs identified are pro-oncogenic, with elevated expression in TNBC. Only a few of them play an anti-tumor role and are downregulated in TNBC. Recent research found that they are all related to clinicopathological characteristics and OS or DFS. Among them, circCAPG[76] (AUC 0.8723), circDNAJC11[108] (AUC 0.658), circSEPT9[112] (AUC 0.711), circRAD18[116] (AUC 0.752), circTADA2A-E6[117] (AUC 08554), and circAHNAK1[119] (AUC 0.72) have a role as prognostic markers for TNBC treatment. In addition, circ 0072309[124] can also be used as a biomarker, but there is currently no research on its relationship with clinicopathological characteristics and survival rate. Circ_0000851 is associated with Ki-67, tumor size, and lymph node metastasis, but the relationship to survival has yet to be elucidated[81]. It is possible that additional circRNAs will be discovered in the future, which could serve as prognostic markers and promising targets for the therapy of TNBC.
CircRNAs associated with TNBC survival prognosis
CircRNAs | Roles | TNBC patients | Expression | Survival | Clinicopathological characteristics | Ref. |
CircCAPG | Oncogenic | 132 | Up | OS | Tumor size, lymph node metastasis and TNM stage | [76] |
CircEGFR | Oncogenic | 38 | Up | OS, DFS | No correlation | [39] |
CircDNAJC11 | Oncogenic | 269 | Up | OS | TNM stage | [108] |
CircFGFR4 | Oncogenic | 60 | Up | OS | Tumor size | [43] |
CircCREIT | Antitumor | 244 | Down | OS | Pathological grade, lymph node metastasis and tumor size | [97] |
CircTBC1D14 | Oncogenic | 237 | Up | OS | Tumor size, Ki67 expression, lymph node metastasis, and distant metastasis | [109] |
CircPTK2 | Oncogenic | 45 | Up | OS | Not analyzed | [51] |
Circ_0000977 | Antitumor | 82 | Down | DFS | Tumor size and age | [110] |
CircWAC | Oncogenic | 90 | Up | OS | No correlation | [57] |
Circ_0044234 | Antitumor | 87 | Down | DFS | Lymph node metastasis, Ki67 expression, and histological grade | [111] |
CircERBB2 | Oncogenic | 82 | Up | OS | TNM stage and lymph node metastasis | [56] |
CircWHSC1 | Oncogenic | 65 | Up | OS | Not analyzed | [38] |
Circ 102229 | Oncogenic | 72 | Up | OS | Tumor size, lymph node metastasis and TNM stage | [61] |
CircNR3C2 | Antitumor | 60 | Down | RFS | Not analyzed | [58] |
CircSEPT9 | Oncogenic | 80 | Up | OS | TNM stage | [112] |
CircUSP42 | Antitumor | 30 | Down | OS, DFS | Lymph node metastasis and clinical stage | [113] |
CircGNB1 | Oncogenic | 222 | Up | OS, DFS | Tumor size and clinical stage | [114] |
Circ 0131242 | Oncogenic | 120 | Up | OS | Tumor size and TNM stages | [115] |
Circ-HER2 | Oncogenic | 59 | Up | OS | Not analyzed | [79] |
CircPGAP3 | Oncogenic | 86 | Up | OS, DFS | Tumor size, lymph node metastasis and TNM stage | [64] |
CircUBE2D2 | Oncogenic | 66 | Up | OS | Not analyzed | [41] |
CircRAD18 | Oncogenic | 126 | Up | OS | T stage, tumor size and clinical stage | [116] |
CircFBXW7 | Antitumor | 473 | Down | OS, DFS | Tumor size and lymph node metastasis | [11] |
CircTADA2A-E6 | Antitumor | 115 | Up | OS, DFS | Lymphatic metastasis and clinical stage | [117] |
Circ 069718 | Oncogenic | 35 | Up | OS | TNM stage, lymph node metastasis | [118] |
CircAHNAK1 | Antitumor | 136 | Down | OS, DFS | Tumor size, lymph node metastasis, and TNM stage | [119] |
CircITCH | Antitumor | 91 | Down | OS | Tumor size, lymph node metastasis and TNM stage | [120] |
CircKIF4A | Oncogenic | 240 | Up | OS, DFS | Tumor size, lymph node metastasis and TNM stage | [121] |
CircPLK1 | Oncogenic | 240 | Up | OS, DFS | Tumor size, lymph node metastasis | [65] |
CircANKS1B | Oncogenic | 165 | Up | OS | Lymph node metastasis and TNM stage | [122] |
CircUBAP2 | Oncogenic | 78 | Up | OS | Tumor size, lymph node metastasis and TNM stage | [52] |
CircEPSTI1 | Oncogenic | 240 | Up | OS, DFS | Tumor size, lymph node metastasis and TNM stage | [67] |
CircGFRA1 | Oncogenic | 222 | Up | OS, DFS | Tumor size, TNM stage, lymph node metastasis and histological grade | [123] |
CircIFI30 | Oncogenic | 78 | Up | OS | Age, histological grade and clinical stage | [63] |
CONCLUSION
Breast cancer is a major life-threatening disease for women around the world and has to be examined in depth. As the most malignant type among them, TNBC deserves wider attention. Scientific and technological development has led to the gradual recognition of circRNA with special properties, which has become a popular area of research in recent years. CircRNA is widely expressed in organisms due to its structural stability. Recent studies indicated that circRNA is not a class of by-products, but a normal component of the body that plays an important regulatory role in a variety of diseases, including tumor. Accumulating evidence suggests that circRNA is related to the survival and prognosis of TNBC and can be used as a biomarker to predict outcomes of the treatment, which opens up another new direction for TNBC therapeutics in the clinic. However, many studies have shown that circRNAs are expressed with spatiotemporal dynamics even within the same tumor, which in turn poses limitations to its use as a therapeutic tool. Currently, there are still many issues that need further research regarding circRNAs: (1) although circRNAs play a significant role in TNBC tumorigenesis and development, our current understanding of their various functions is still limited; (2) in addition to studying the specific mechanisms of circRNA involvement in TNBC tumorigenesis and development, it is important to translate useful experimental findings into clinical products for the benefit of breast cancer patients; and (3) research on circRNA still focuses on sponge function, while the mechanism and function of circRNA as a translation template or binding protein are still relatively unknown. This review briefly explained the biosynthetic mechanism and functions of circRNA. We also described several major biological events in which circRNA is involved for the progression of TNBC, and summarized current information on circRNAs in relation to the survival prognosis of TNBC. This article did not explain how circRNA is degraded or about its regulatory relationship with parental genes. Additionally, the summarized biological events are not comprehensive, but representative of selected hot topics at present.
DECLARATIONS
Authors’ contributions
Writing-original draft: Xu A
Writing-review and editing: Zhu L
Collect some literature: Yao C
Providing review ideas and guidance: Zhou W, Guan Z
Availability of data and materials
Not applicable.
Financial support and sponsorship
This research was supported by the Guangdong Basic and Applied Basic Research Foundation (Nos. 2021B1515120053, 2020A1515010185).
Conflict of interests
All authors declared that there are no conflicts of interest.
Ethical approval and consent to participate
Not applicable.
Consent for publication
Not applicable.
Copyright
© The Author(s) 2024.
REFERENCES
1. Sung H, Ferlay J, Siegel RL, et al. Global cancer statistics 2020: GLOBOCAN estimates of incidence and mortality worldwide for 36 cancers in 185 countries. CA Cancer J Clin 2021;71:209-49.
2. Perou CM, Sørlie T, Eisen MB, et al. Molecular portraits of human breast tumours. Nature 2000;406:747-52.
3. Ensenyat-mendez M, Llinàs-arias P, Orozco JIJ, et al. Current triple-negative breast cancer subtypes: dissecting the most aggressive form of breast cancer. Front Oncol 2021;11:681476.
4. Yin L, Duan J, Bian X, Yu S. Triple-negative breast cancer molecular subtyping and treatment progress. Breast Cancer Res 2020;22:61.
5. Asleh K, Riaz N, Nielsen TO. Heterogeneity of triple negative breast cancer: Current advances in subtyping and treatment implications. J Exp Clin Cancer Res 2022;41:265.
6. Xie J, Ye F, Deng X, et al. Circular RNA: a promising new star of vaccine. J Transl Int Med 2023;11:372-81.
7. Vo JN, Cieslik M, Zhang Y, et al. The landscape of circular RNA in cancer. Cell 2019;176:869-81.e13.
8. Zeng Y, Zou Y, Gao G, et al. The biogenesis, function and clinical significance of circular RNAs in breast cancer. Cancer Bio Med 2021;19:14-29.
9. Hansen TB, Jensen TI, Clausen BH, et al. Natural RNA circles function as efficient microRNA sponges. Nature 2013;495:384-8.
10. Zeng Y, Du W, Huang Z, et al. Hsa_circ_0060467 promotes breast cancer liver metastasis by complexing with eIF4A3 and sponging miR-1205. Cell Death Discov 2023;9:153.
11. Ye F, Gao G, Zou Y, et al. circFBXW7 inhibits malignant progression by sponging miR-197-3p and encoding a 185-aa protein in triple-negative breast cancer. Mol Ther Nucleic Acids 2019;18:88-98.
12. Hsu MT, Coca-Prados M. Electron microscopic evidence for the circular form of RNA in the cytoplasm of eukaryotic cells. Nature 1979;280:339-40.
13. Cocquerelle C, Mascrez B, Hétuin D, Bailleul B. Mis-splicing yields circular RNA molecules. FASEB J 1993;7:155-60.
14. Chen L, Wang C, Sun H, et al. The bioinformatics toolbox for circRNA discovery and analysis. Brief Bioinform 2021;22:1706-28.
15. Memczak S, Jens M, Elefsinioti A, et al. Circular RNAs are a large class of animal RNAs with regulatory potency. Nature 2013;495:333-8.
16. Haynes B, Sarma A, Nangia-makker P, Shekhar MP. Breast cancer complexity: implications of intratumoral heterogeneity in clinical management. Cancer Metastasis Rev 2017;36:547-55.
17. Zhang C, Zhang B. RNA therapeutics: updates and future potential. Sci China Life Sci 2023;66:12-30.
18. Rong Z, Xu J, Shi S, et al. Circular RNA in pancreatic cancer: a novel avenue for the roles of diagnosis and treatment. Theranostics 2021;11:2755-69.
19. Liu CX, Chen LL. Circular RNAs: characterization, cellular roles, and applications. Cell 2022;185:2016-34.
20. Li X, Zhang JL, Lei YN, et al. Linking circular intronic RNA degradation and function in transcription by RNase H1. Sci China Life Sci 2021;64:1795-809.
21. Conn VM, Hugouvieux V, Nayak A, et al. A circRNA from SEPALLATA3 regulates splicing of its cognate mRNA through R-loop formation. Nature Plants 2017;3:17053.
22. Jeck WR, Sorrentino JA, Wang K, et al. Circular RNAs are abundant, conserved, and associated with ALU repeats. RNA 2013;19:141-57.
23. Zhang X, Wang H, Zhang Y, Lu X, Chen L, Yang L. Complementary sequence-mediated exon circularization. Cell 2014;159:134-47.
24. Conn S, Pillman K, Toubia J, et al. The RNA binding protein quaking regulates formation of circRNAs. Cell 2015;160:1125-34.
25. Errichelli L, Dini Modigliani S, Laneve P, et al. FUS affects circular RNA expression in murine embryonic stem cell-derived motor neurons. Nat Commun 2017;8:14741.
26. Ashwal-fluss R, Meyer M, Pamudurti N, et al. circRNA biogenesis competes with pre-mRNA splicing. Molecular Cell 2014;56:55-66.
27. Guo Y, Yang J, Huang Q, et al. Circular RNAs and their roles in head and neck cancers. Mol Cancer 2019;18:44.
28. Zhang Y, Zhang X, Chen T, et al. Circular intronic long noncoding RNAs. Mol Cell 2013;51:792-806.
29. Hoque P, Romero B, Akins RE, Batish M. Exploring the multifaceted biologically relevant roles of circRNAs: from regulation, translation to biomarkers. Cells 2023;12:2813.
30. Xin R, Gao Y, Gao Y, et al. isoCirc catalogs full-length circular RNA isoforms in human transcriptomes. Nat Commun 2021;12:266.
31. Chiang TW, Jhong SE, Chen YC, Chen CY, Wu WS, Chuang TJ. FL-circAS: an integrative resource and analysis for full-length sequences and alternative splicing of circular RNAs with nanopore sequencing. Nucleic Acids Res 2024;52:D115-23.
33. Tay Y, Rinn J, Pandolfi PP. The multilayered complexity of ceRNA crosstalk and competition. Nature 2014;505:344-52.
34. Verduci L, Strano S, Yarden Y, Blandino G. The circ RNA-micro RNA code: emerging implications for cancer diagnosis and treatment. Mol Oncol 2019;13:669-80.
35. Zou Y, Zheng S, Deng X, et al. The role of circular RNA CDR1as/ciRS-7 in regulating tumor microenvironment: a pan-cancer analysis. Biomolecules 2019;9:429.
36. Sang M, Meng L, Liu S, et al. Circular RNA ciRS-7 maintains metastatic phenotypes as a ceRNA of miR-1299 to target MMPs. Mol Cancer Res 2018;16:1665-75.
37. Li Y, Jiang B, Zeng L, et al. Adipocyte-derived exosomes promote the progression of triple-negative breast cancer through circCRIM1-dependent OGA activation. Environ Res 2023;239:117266.
38. Ding L, Xie Z. CircWHSC1 regulates malignancy and glycolysis by the miR-212-5p/AKT3 pathway in triple-negative breast cancer. Exp Mol Pathol 2021;123:104704.
39. Ma J, Chen C, Fan Z, et al. CircEGFR reduces the sensitivity of pirarubicin and regulates the malignant progression of triple-negative breast cancer via the miR-1299/EGFR axis. Int J Biol Macromol 2023;244:125295.
40. Huang S, Xie J, Lei S, Fan P, Zhang C, Huang Z. CircDUSP1 regulates tumor growth, metastasis, and paclitaxel sensitivity in triple-negative breast cancer by targeting miR-761/DACT2 signaling axis. Mol Carcinog 2023;62:450-63.
41. Dou D, Ren X, Han M, et al. CircUBE2D2 (hsa_circ_0005728) promotes cell proliferation, metastasis and chemoresistance in triple-negative breast cancer by regulating miR-512-3p/CDCA3 axis. Cancer Cell Int 2020;20:454.
42. He ZY, Zhuo RG, Yang SP, et al. CircNCOR1 regulates breast cancer radiotherapy efficacy by regulating CDK2 via hsa-miR-638 binding. Cell Signal 2023;109:110787.
43. Wang F, Lu Q, Yu H, Zhang XM. The circular RNA circFGFR4 facilitates resistance to anti-PD-1 of triple-negative breast cancer by targeting the miR-185-5p/CXCR4 axis. Cancer Manag Res 2023;15:825-35.
44. Wang Z, Li Y, Yang J, et al. Circ-TRIO promotes TNBC progression by regulating the miR-432-5p/CCDC58 axis. Cell Death Dis 2022;13:776.
45. He Q, Hao Q, Wu Y, Vadgama JV, Jiang Y. CircRAD54L2 promotes triple-negative breast cancer progression by regulating the miR-888 family/PDK1 axis. Life Sci 2023;312:121128.
46. Shao G, Fan X, Zhang P, Liu X, Huang L, Ji S. Circ_0004676 exacerbates triple-negative breast cancer progression through regulation of the miR-377-3p/E2F6/PNO1 axis. Cell Biol Toxicol 2023;39:2183-205.
47. Zan X, Li W, Wang G, et al. Circ-CSNK1G1 promotes cell proliferation, migration, invasion and glycolysis metabolism during triple-negative breast cancer progression by modulating the miR-28-5p/LDHA pathway. Reprod Biol Endocrinol 2022;20:138.
48. Gong G, She J, Fu D, Zhen D, Zhang B. CircUBR5 acts as a ceRNA for miR-1179 to up-regulate UBR5 and to promote malignancy of triple-negative breast cancer. Am J Cancer Res 2022;12:2539-57.
49. Cui S, Zhang Y, Xing L, Li R, Piao Y, Liu H. Circular RNA dehydrodolichyl diphosphate synthase facilitated triple-negative breast cancer progression via miR-362-3p/DDX5 axis. Environ Toxicol 2022;37:1483-94.
50. Wang X, Song H, Fang L, Wu T. EIF4A3-mediated circPRKCI expression promotes triple-negative breast cancer progression by regulating WBP2 and PI3K/AKT signaling pathway. Cell Death Discov 2022;8:92.
51. Wang M, Chen D, Zhang H, Luo C. Circular RNA circPTK2 modulates migration and invasion via miR-136/NFIB signaling on triple-negative breast cancer cells in vitro. Inflamm Res 2022;71:409-21.
52. Wang L, Yang X, Zhou F, Sun X, Li S. Circular RNA UBAP2 facilitates the cisplatin resistance of triple-negative breast cancer via microRNA-300/anti-silencing function 1B histone chaperone/PI3K/AKT/mTOR axis. Bioengineered 2022;13:7197-208.
53. Maimaiti Y, Zhang N, Zhang Y, Zhou J, Song H, Wang S. CircFAM64A enhances cellular processes in triple-negative breast cancer by targeting the miR-149-5p/CDT1 axis. Environ Toxicol 2022;37:1081-92.
54. Li J, Gao X, Zhang Z, et al. CircCD44 plays oncogenic roles in triple-negative breast cancer by modulating the miR-502-5p/KRAS and IGF2BP2/Myc axes. Mol Cancer 2021;20:138.
55. Xing Z, Wang R, Wang X, et al. CircRNA circ-PDCD11 promotes triple-negative breast cancer progression via enhancing aerobic glycolysis. Cell Death Discov 2021;7:218.
56. Huang Y, Zheng S, Lin Y, Ke L. Circular RNA circ-ERBB2 elevates the warburg effect and facilitates triple-negative breast cancer growth by the microRNA 136-5p/pyruvate dehydrogenase kinase 4 axis. Mol Cell Biol 2021;41:e00609-20.
57. Wang L, Zhou Y, Jiang L, et al. CircWAC induces chemotherapeutic resistance in triple-negative breast cancer by targeting miR-142, upregulating WWP1 and activating the PI3K/AKT pathway. Mol Cancer 2021;20:43.
58. Fan Y, Wang J, Jin W, et al. CircNR3C2 promotes HRD1-mediated tumor-suppressive effect via sponging miR-513a-3p in triple-negative breast cancer. Mol Cancer 2021;20:25.
59. Yang SJ, Wang DD, Zhong SL, et al. Tumor-derived exosomal circPSMA1 facilitates the tumorigenesis, metastasis, and migration in triple-negative breast cancer (TNBC) through miR-637/Akt1/β-catenin (cyclin D1) axis. Cell Death Dis 2021;12:420.
60. Wang X, Xue B, Zhang Y, Guo G, Duan X, Dou D. Up-regulated circBACH2 contributes to cell proliferation, invasion, and migration of triple-negative breast cancer. Cell Death Dis 2021;12:412.
61. Du C, Zhang J, Zhang L, Zhang Y, Wang Y, Li J. Hsa_circRNA_102229 facilitates the progression of triple-negative breast cancer via regulating the miR-152-3p/PFTK1 pathway. J Gene Med 2021;23:e3365.
62. Li H, Xu W, Xia Z, et al. Hsa_circ_0000199 facilitates chemo-tolerance of triple-negative breast cancer by interfering with miR-206/613-led PI3K/Akt/mTOR signaling. Aging 2021;13:4522-51.
63. Xing L, Yang R, Wang X, et al. The circRNA circIFI30 promotes progression of triple-negative breast cancer and correlates with prognosis. Aging 2020;12:10983-1003.
64. He D, Yang X, Kuang W, Huang G, Liu X, Zhang Y. The novel circular RNA circ-PGAP3 promotes the proliferation and invasion of triple negative breast cancer by regulating the miR-330-3p/Myc axis. Onco Targets Ther 2020;13:10149-59.
65. Kong Y, Yang L, Wei W, et al. CircPLK1 sponges miR-296-5p to facilitate triple-negative breast cancer progression. Epigenomics 2019;11:1163-76.
66. Wu S, Lu J, Zhu H, et al. A novel axis of circKIF4A-miR-637-STAT3 promotes brain metastasis in triple-negative breast cancer. Cancer Lett 2024;581:216508.
67. Chen B, Wei W, Huang X, et al. circEPSTI1 as a prognostic marker and mediator of triple-negative breast cancer progression. Theranostics 2018;8:4003-15.
68. Dudekula DB, Panda AC, Grammatikakis I, De S, Abdelmohsen K, Gorospe M. CircInteractome: a web tool for exploring circular RNAs and their interacting proteins and microRNAs. RNA Biology 2016;13:34-42.
69. Pamudurti NR, Patop IL, Krishnamoorthy A, et al. circMbl functions in cis and in trans to regulate gene expression and physiology in a tissue-specific fashion. Cell Rep 2022;39:110740.
70. Luo P, Gong Y, Weng J, et al. CircKIF4A combines EIF4A3 to stabilize SDC1 expression to activate c-src/FAK and promotes TNBC progression. Cell Signal 2023;108:110690.
71. Gao H, Tuluhong D, Li X, et al. CircSNX25 mediated by SP1 promotes the carcinogenesis and development of triple-negative breast cancer. Cell Signal 2023;109:110776.
72. Turco C, Esposito G, Iaiza A, et al. MALAT1-dependent hsa_circ_0076611 regulates translation rate in triple-negative breast cancer. Commun Biol 2022;5:598.
73. Song X, Chen B, Liang Y, et al. CircEIF3H-IGF2BP2-HuR scaffold complex promotes TNBC progression via stabilizing HSPD1/RBM8A/G3BP1 mRNA. Cell Death Discov 2022;8:261.
74. Abe N, Matsumoto K, Nishihara M, et al. Rolling circle translation of circular RNA in living human cells. Sci Rep 2015;5:16435.
75. Meyer K, Patil D, Zhou J, et al. 5′ UTR m6A Promotes cap-independent translation. Cell 2015;163:999-1010.
76. Song R, Guo P, Ren X, et al. A novel polypeptide CAPG-171aa encoded by circCAPG plays a critical role in triple-negative breast cancer. Mol Cancer 2023;22:104.
77. Li Y, Wang Z, Su P, et al. circ-EIF6 encodes EIF6-224aa to promote TNBC progression via stabilizing MYH9 and activating the Wnt/beta-catenin pathway. Mol Ther 2022;30:415-30.
78. Wang X, Jian W, Luo Q, Fang L. CircSEMA4B inhibits the progression of breast cancer by encoding a novel protein SEMA4B-211aa and regulating AKT phosphorylation. Cell Death Dis 2022;13:794.
79. Li J, Ma M, Yang X, et al. Circular HER2 RNA positive triple negative breast cancer is sensitive to Pertuzumab. Mol Cancer 2020;19:142.
80. Hao R, Zhang L, Si Y, et al. A novel feedback regulated loop of circRRM2-IGF2BP1-MYC promotes breast cancer metastasis. Cancer Cell Int 2023;23:54.
81. Ji C, Zhu L, Fang L. Hsa_circ_0000851 promotes PDK1/p-AKT-mediated cell proliferation and migration by regulating miR-1183 in triple-negative breast cancer. Cell Signal 2023;101:110494.
82. Liu P, Wang Z, Ou X, et al. The FUS/circEZH2/KLF5/ feedback loop contributes to CXCR4-induced liver metastasis of breast cancer by enhancing epithelial-mesenchymal transition. Mol Cancer 2022;21:198.
83. Ju C, Zhou M, Du D, et al. EIF4A3-mediated circ_0042881 activates the RAS pathway via miR-217/SOS1 axis to facilitate breast cancer progression. Cell Death Dis 2023;14:559.
84. Huang X, Tan W, Liu Z, et al. EIF4A3-induced circZFAND6 promotes breast cancer proliferation and metastasis through the miR-647/FASN axis. Life Sci 2023;324:121745.
85. Zheng W, Wang X, Yu Y, Ji C, Fang L. CircRNF10-DHX15 interaction suppressed breast cancer progression by antagonizing DHX15-NF-κB p65 positive feedback loop. Cell Mol Biol Lett 2023;28:34.
86. Meng L, Chang S, Sang Y, et al. Circular RNA circCCDC85A inhibits breast cancer progression via acting as a miR-550a-5p sponge to enhance MOB1A expression. Breast Cancer Res 2022;24:1.
88. Safi A, Saberiyan M, Sanaei M, et al. The role of noncoding RNAs in metabolic reprogramming of cancer cells. Cell Mol Biol Lett 2023;28:37.
89. Cai Z, Hu Y, Liao K, Li H, Chen D, Ju H. Circular RNAs: emerging regulators of glucose metabolism in cancer. Cancer Lett 2023;552:215978.
90. Ma W, Sun X, Zhang S, Chen Z, Yu J. Circ_0039960 regulates growth and Warburg effect of breast cancer cells via modulating miR-1178/PRMT7 axis. Mol Cell Probes 2022;64:101829.
91. Dou D, Ren X, Han M, et al. Circ_0008039 supports breast cancer cell proliferation, migration, invasion, and glycolysis by regulating the miR-140-3p/SKA2 axis. Mol Oncol 2021;15:697-709.
92. Cao L, Wang M, Dong Y, et al. Circular RNA circRNF20 promotes breast cancer tumorigenesis and Warburg effect through miR-487a/HIF-1α/HK2. Cell Death Dis 2020;11:145.
93. Tian J, Liu S, Yu C, Wu L, Wang L. The role of non-coding RNAs in breast cancer drug resistance. Front Oncol 2021;11:702082.
94. Tang AH, Hoefer RA, Guye ML, Bear HD. Persistent EGFR/K-RAS/SIAH pathway activation drives chemo-resistance and early tumor relapse in triple-negative breast cancer. Cancer Drug Resist 2022;5:691-702.
95. Xia M, Zu X, Chen Z, Wen G, Zhong J. Noncoding RNAs in triple negative breast cancer: mechanisms for chemoresistance. Cancer Lett 2021;523:100-10.
96. Misir S, Ozer Yaman S, Petrović N, Sumer C, Hepokur C, Aliyazicioglu Y. circRNAs in drug resistance of breast cancer. Oncol Res 2022;30:157-72.
97. Wang X, Chen T, Li C, et al. CircRNA-CREIT inhibits stress granule assembly and overcomes doxorubicin resistance in TNBC by destabilizing PKR. J Hematol Oncol 2022;15:122.
98. Wang X, Yao Y, Jin M. Circ-0001068 is a novel biomarker for ovarian cancer and inducer of PD1 expression in T cells. Aging 2020;12:19095-106.
99. Wang J, Zhao X, Wang Y, et al. circRNA-002178 act as a ceRNA to promote PDL1/PD1 expression in lung adenocarcinoma. Cell Death Dis 2020;11:32.
100. Xu Y, Zhao J, Gao C, et al. Hsa_circ_0136666 activates Treg-mediated immune escape of colorectal cancer via miR-497/PD-L1 pathway. Cell Signal 2021;86:110095.
101. Gao C, Xu Y, J Qi L, Bao YF, Zhang L, Zheng L. CircRNA VIM silence synergizes with sevoflurane to inhibit immune escape and multiple oncogenic activities of esophageal cancer by simultaneously regulating miR-124/PD-L1 axis. Cell Biol Toxicol 2022;38:825-45.
102. Fu X, Sun G, Tu S, et al. Hsa_circ_0046523 mediates an immunosuppressive tumor microenvironment by regulating MiR-148a-3p/PD-L1 axis in pancreatic cancer. Front Oncol 2022;12:877376.
103. Yang Z, Chen W, Wang Y, Qin M, Ji Y. CircKRT1 drives tumor progression and immune evasion in oral squamous cell carcinoma by sponging miR-495-3p to regulate PDL1 expression. Cell Biol Int 2021;45:1423-35.
104. Luo Y, Tang W, Xiang S, Feng J, Zu X. Non-coding RNAs in breast cancer: implications for programmed cell death. Cancer Lett 2022;550:215929.
105. Zheng Y, Ren S, Zhang Y, et al. Circular RNA circWWC3 augments breast cancer progression through promoting M2 macrophage polarization and tumor immune escape via regulating the expression and secretion of IL-4. Cancer Cell Int 2022;22:264.
106. Lu C, Shi W, Hu W, et al. Endoplasmic reticulum stress promotes breast cancer cells to release exosomes circ_0001142 and induces M2 polarization of macrophages to regulate tumor progression. Pharmacol Res 2022;177:106098.
107. Huang X, Song C, Zhang J, Zhu L, Tang H. Circular RNAs in breast cancer diagnosis, treatment and prognosis. Oncol Res 2024;32:241-9.
108. Wang B, Chen H, Deng Y, et al. CircDNAJC11 interacts with TAF15 to promote breast cancer progression via enhancing MAPK6 expression and activating the MAPK signaling pathway. J Transl Med 2023;21:186.
109. Liu Y, Liu Y, He Y, et al. Hypoxia-induced FUS-circTBC1D14 stress granules promote autophagy in TNBC. Adv Sci 2023;10:2204988.
110. Darbeheshti F, Mansoori Y, Azizi-tabesh G, et al. Evaluation of circ_0000977-mediated regulatory network in breast cancer: a potential discriminative biomarker for triple-negative tumors. Biochem Genet 2023;61:1487-508.
111. Darbeheshti F, Zokaei E, Mansoori Y, et al. Circular RNA hsa_circ_0044234 as distinct molecular signature of triple negative breast cancer: a potential regulator of GATA3. Cancer Cell Int 2021;21:312.
112. Zheng X, Huang M, Xing L, et al. The circRNA circSEPT9 mediated by E2F1 and EIF4A3 facilitates the carcinogenesis and development of triple-negative breast cancer. Mol Cancer 2020;19:73.
113. Yu J, Shen W, Xu J, Gong B, Gao B, Zhu J. circUSP42 is downregulated in triple-negative breast cancer and associated with poor prognosis. Technol Cancer Res Treat 2020;19:153303382095082.
114. Liu P, Zou Y, Li X, et al. circGNB1 facilitates triple-negative breast cancer progression by regulating miR-141-5p-IGF1R axis. Front Genet 2020;11:193.
115. Li Y, Shi P, Zheng T, Ying Z, Jiang D. Circular RNA hsa_circ_0131242 promotes triple-negative breast cancer progression by sponging hsa-miR-2682. Onco Targets Ther 2020;13:4791-8.
116. Zou Y, Zheng S, Xiao W, et al. circRAD18 sponges miR-208a/3164 to promote triple-negative breast cancer progression through regulating IGF1 and FGF2 expression. Carcinogenesis 2019;40:1469-79.
117. Xu J, Shao C, Wang X, et al. circTADA2As suppress breast cancer progression and metastasis via targeting miR-203a-3p/SOCS3 axis. Cell Death Dis 2019;10:175.
118. Zhang J, Xu HD, Xing XJ, Liang ZT, Xia ZH, Zhao Y. CircRNA_069718 promotes cell proliferation and invasion in triple-negative breast cancer by activating Wnt/β-catenin pathway. Eur Rev Med Pharmacol Sci 2019;23:5315-22.
119. Xiao W, Zheng S, Zou Y, et al. CircAHNAK1 inhibits proliferation and metastasis of triple-negative breast cancer by modulating miR-421 and RASA1. Aging 2019;11:12043-56.
120. Wang ST, Liu LB, Li XM, et al. Circ-ITCH regulates triple-negative breast cancer progression through the Wnt/β-catenin pathway. Neoplasma 2019;66:232-9.
121. Tang H, Huang X, Wang J, et al. circKIF4A acts as a prognostic factor and mediator to regulate the progression of triple-negative breast cancer. Mol Cancer 2019;18:23.
122. Zeng K, He B, Yang BB, et al. The pro-metastasis effect of circANKS1B in breast cancer. Mol Cancer 2018;17:160.
123. He R, Liu P, Xie X, et al. circGFRA1 and GFRA1 act as ceRNAs in triple negative breast cancer by regulating miR-34a. J Exp Clin Cancer Res 2017;36:145.
Cite This Article
Export citation file: BibTeX | RIS
OAE Style
Xu A, Zhu L, Yao C, Zhou W, Guan Z. The therapeutic potential of circular RNA in triple-negative breast cancer. Cancer Drug Resist 2024;7:13. http://dx.doi.org/10.20517/cdr.2023.141
AMA Style
Xu A, Zhu L, Yao C, Zhou W, Guan Z. The therapeutic potential of circular RNA in triple-negative breast cancer. Cancer Drug Resistance. 2024; 7: 13. http://dx.doi.org/10.20517/cdr.2023.141
Chicago/Turabian Style
Xu, Aiqi, Lewei Zhu, Chengcai Yao, Wen Zhou, Ziyun Guan. 2024. "The therapeutic potential of circular RNA in triple-negative breast cancer" Cancer Drug Resistance. 7: 13. http://dx.doi.org/10.20517/cdr.2023.141
ACS Style
Xu, A.; Zhu L.; Yao C.; Zhou W.; Guan Z. The therapeutic potential of circular RNA in triple-negative breast cancer. Cancer Drug Resist. 2024, 7, 13. http://dx.doi.org/10.20517/cdr.2023.141
About This Article
Special Issue
Copyright
Data & Comments
Data
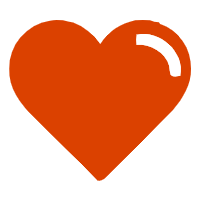

Comments
Comments must be written in English. Spam, offensive content, impersonation, and private information will not be permitted. If any comment is reported and identified as inappropriate content by OAE staff, the comment will be removed without notice. If you have any queries or need any help, please contact us at support@oaepublish.com.